Net Zero Carbon Supplementary Planning Document
5. Policy NZC2(B) – Zero or Low Carbon Energy Sources
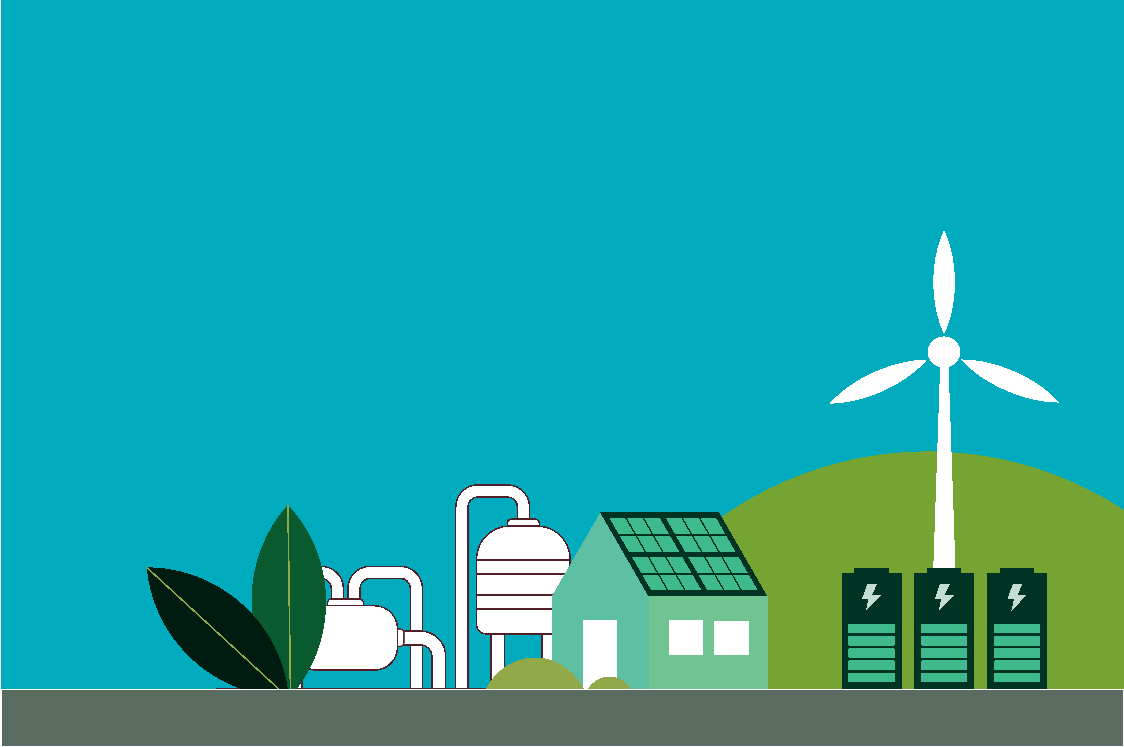
5.1 All measures that relate to an improvement in the carbon intensity of the supply of energy are covered under Policy NZC2(B): Zero or Low Carbon Energy Sources and Zero Carbon Ready Technology.
Policy NZC2(B): Zero or Low Carbon Energy Sources and Zero Carbon Ready Technology
New development of one or more new dwellings (C3 or C4 use class) and/or 1,000sqm or more of new non-residential floorspace, hotels (C1 use class), or residential institutions (C2 use class) should demonstrate through an energy statement that additional renewable, zero and low carbon energy technologies have been provided on-site* to achieve the carbon reductions required by Policy NZC1 and achieve on-site net zero regulated operational carbon.
Where full compliance is not feasible or viable having regard to the type of development involved and its design, proposals must:
- demonstrate through the energy statement that additional renewable, zero and low carbon energy technologies have been provided to the greatest extent feasible and viable.
- incorporate 'zero carbon ready' (as opposed to immediately providing 'low/zero carbon') technologies.
*this may include off site existing or planned zero, low carbon or renewable energy generation or heat network provision where there is a direct off-grid connection to the development which has capacity to serve the development.
5.2 The policy requires the following provision of low-carbon and renewable energy sources to achieve the required improvements on the baseline:
Table 6: Summary of Policy NZC2(B) requirements for low-carbon energy supply by building type, and rationale.
Use type |
Baseline measure |
Baseline edition of Building Regs |
Required improvement on baseline |
New development of one or more dwellings (C3 or C4 use class) |
Part L 2021 Target Emission Rate (TER) |
Part L 2021 |
63% minimum 100% where feasible in combination with the fabric efficiency improvements already achieved under Policy NZC2(A). |
New development of 1,000sqm or more of new non-residential floorspace, hotels (C1 use class) or residential institutions (C2 use class). |
Part L 2013 Target Emission Rate |
Part L 2013 |
35% minimum 100% where feasible in combination with the fabric efficiency improvements already achieved under Policy NZC2(A). |
Where it is not feasible to meet the applicable target noted above, proposals must demonstrate that carbon reductions to the greatest extent feasible have been pursued. This should be identified in the Energy Statement. |
5.3 Measures that can be considered to contribute towards the requirements of Policy NZC2(B) include any measure that is low-carbon
in comparison to the Building Regulations baseline for that type of energy use, such as:
- Direct electric heating, as grid electricity has a lower carbon factor per kWh than fossil gas (but only recommended currently alongside an additional renewable energy source such as solar panels or a heat pump).
- Heat networks supplied by fossil fuel free sources, including waste heat.
- Solar, hydro or wind energy generated on site, as this is zero- carbon and thus lower-carbon than grid electricity.
- Biomass or biogas, within strict criteria whereby the fuel source is sustainably managed and/or is a waste product that would otherwise create CO2 in its decay if otherwise disposed.
- Heat pumps, as these deliver approximately 2.5–4 units of renewable heat for every 1 unit they consume in grid electricity (depending on the Coefficient of Performance that is achieved which varies by type and quality of heat pump).
- Energy storage (electrical or thermal), as this can, when combined with clean energy generation, increase the proportion of the clean energy generated on-site that is consumed at the development. Without energy storage, developments with large amounts of renewable energy generation on site may have to import higher-carbon energy from the grid at times of low generation, and either discard or export lower-carbon energy to the grid at times of high generation and low usage, whereby some of that exported energy would be lost in distribution before that energy can be consumed by another user).
5.4 Please note that some of the low carbon energy supply measures noted above have an element of 'energy efficiency' and therefore can, in non-domestic applications be categorised as 'energy efficiency' measures, as previously noted in the guidance for Policy NZC2(A) and Table 3.
5.5 Tables 7–18 below provide a summary of the available types of renewable and low-carbon energy supply measures and their general suitability to different situations.
Table 7:
Air source heat pumps
What is it?
An air source heat pump (ASHP) is able to heat and cool a building through recycling heat. Similar to a fridge but in reverse, an ASHP takes heat from outside air and transfers it to an internal heating system. This can be used for heating and hot water. Some heat pumps can reverse the system to provide a cooling function. Heat pumps provide heat efficiently at lower temperatures, meaning they are paired effectively with low temperature heating systems such as underfloor heating systems, which usually have a flow temperature of 35°C compared to <70°C demanded by gas boiler fed radiators – if underfloor heating is not possible then large radiators should be installed. Since ASHPs provide heat at low temperatures, it is important the building is well insulated so that less heat is required and can be retained.
How efficient is it?
Heat pumps are significantly more efficient than direct electric or combustion-based systems because for one unit of electricity used, multiple units of heat are generated. ASHPs typically have a coefficient of performance (COP) above 3, meaning they are > 300% efficient. The efficiency of the technology will decrease as the disparity between outdoor temperature and desired indoor temperature increases, however, the seasonal efficiencies of modern domestic units when properly installed, typically exceed 350%. Installing an ASHP will make compliance with Policy NZC2(B) easier to achieve than less efficient heating systems.
Location and space requirements
The most important element for the location of an ASHP is to ensure steady air flow. To enable this any plants, walls or objects should be placed at least 1 metre away and refer to relevant product installation guidance. The unit should be located immediately outside your home and ideally at the back of the building where easy access is available for maintenance. Placing the unit in a location that requires minimal insulated pipework is important to mitigate heat loss. Where there is insufficient outdoor ground space, ASHPs can be mounted on flat roofs, which can also reduce noise pollution to neighbouring buildings.
Things to be aware of (e.g. noise and visual impact)
Without appropriate acoustic measures, some ASHPs can create noise pollution, for which mitigation could be required for planning compliance. Noise should be a consideration in the design process and can be addressed by selecting a quieter model, siting the ASHP unit away from noise-sensitive receptors such as bedroom windows or addressing the noise at the receptor such as enhanced acoustically rated windows. Encasing an ASHP with an acoustic enclosure can also mitigate noise pollution risks, whilst retaining air flow and not requiring much more additional outdoor space.
Appropriate maintenance of all heat pump units should be carried out to ensure that debris and dust does not build up in filters and the fan, which would subsequently limit efficiency.
Suitability/applicability across schemes
For new build development that must comply with Policy NZC2(B), ASHPs are suitable for the majority of building typologies, since they can be mounted outside the building on the ground or a roof. For most 2- and 3-storey residential typologies, sufficient ground space should be available for ground-mounted units, whilst flatted blocks should be able to install units on the roof. However, roof-mounted units may reduce roof space available for on-site solar PV electricity generation.
In some cases, such as large-scale new schemes, a heat network of heat pumps may be more suitable than individual units. This is explained in the 'District heating' section.
Table 8:
Ground/water source heat pumps
What is it?
A ground source heat pump (GSHP) and a water source heat pump (WSHP) will operate in the same way as an ASHP in terms of heat transfer from outside to inside. However, a GSHP extracts heat from the ground through boreholes and pipework, whereas a WSHP takes heat from bodies of water such as rivers, boreholes and lakes. Flow temperatures are low and similar to ASHP is effectively paired with underfloor heating or requires larger radiators. Both types of heat pump are also able to provide cooling.
How efficient is it?
The efficiency of a GSHP and WSHP is likely to be superior to that of most ASHP units over the course of a year, up to a COP of 4. This is because ground temperatures are more consistent due to higher heat retention, whereas an ASHP is vulnerable to fluctuations in air temperature.
A WSHP is even more efficient than a GSHP since water is an excellent thermal conductor and temperatures tend to be more stable than both air and ground temperatures.
Location and space requirements
A GSHP often requires a large outdoor space for horizontal channels or a deep vertical borehole.
GSHP can also be integrated with structural foundations, e.g., ‘thermal piling’. Where a building already needs to invest in extensive foundations or deep piling, this may present an opportunity to maximise the benefits of this investment by integrating GSHP to these.
A WSHP requires a large body of water to extract sufficient heat (which can be surface water, or groundwater again via a borehole). If a WSHP is installed in a body of water too small, excessive heat could be removed and reduce the temperature to a level where freezing may occur.
The main units of a GSHP and WSHP can be located inside the building since the pipework collects the heat and therefore does not require air flow like an ASHP.
Applicants may find it helpful to refer to guidance from the GSHP Association which offers standards for vertical boreholes, horizontal exchanges, thermal piles and other guidance.
Things to be aware of (e.g. noise and visual impact)
Environmental constraints may limit where WSHPs can be installed. The same applies for GSHP as boreholes can have a negative impact on archaeology. The impact on ecology should be considered for both types of heat pumps.
Suitability/applicability across scheme
The suitability and applicability of GSHPs and WSHPs is not as widespread as of ASHPs because they both require specific settings to be feasible.
Table 9:
Domestic hot water storage
What is it?
Domestic hot water storage generally consists of a cylinder that stores pre-heated water for instant use throughout a house. Cylinder sizes can range from 50 – 500 litres, but 200 litres should be sufficient for an average four- person family. Heat pumps need cylinders with heat exchangers to deliver hot water.
How efficient is it?
The technology increases overall heating efficiency as excess heated water is stored in the tank for later use. Storage tanks reduce water wastage, whilst ensuring hot water is immediately available at all times.
Combining storage tanks with solar thermal energy generation is an efficient combination, whereby the heated water goes straight into the storage tank. This storage of solar renewable energy as hot water improves overall efficiency.
Location and space requirements
Suitable location required, such as an airing cupboard. Significant space around the unit is not necessary but flats may struggle to accommodate sufficient space.
Things to be aware of (e.g. noise and visual impact)
Sufficient insulation of the hot water tank and pipework is essentially for efficiency maximisation, particularly for piping that travels long distances through the building. This is also an important measure to avoid increasing the risk of space overheating in summer.
In accordance with the UK Health and Safety Executive, domestic hot water must be heated to and stored at a temperature of >60°C (amongst other storage and control measures) in order to prevent risk of exposure to Legionnaire's disease in residential and non-residential buildings. Heat pumps typically maintain a temperature of up to 55°C but comply with the requirement through a cleansing cycle that reaches 60°C.
Suitability/applicability across schemes
More suitable for larger homes where there is more demand for hot water but remain suitable in flatted schemes too, although issues could be apparent for space and location requirements.
Table 10:
Heat recovery
What is it?
The two primary mechanisms for heat recovery in buildings are Mechanical Ventilation with Heat Recovery (MVHR) and Waste Water Heat Recovery (WWHR).
MVHR uses a heat exchanger to recover heat from 'used' or extracted air to pre-heat 'fresh' air to be supplied to the dwelling. Additionally, these systems improve indoor air quality by maintaining fresh air and removing stagnant air. WWHR operates in a similar way, with heat from wastewater (e.g. used shower or bath water) being used to pre-heat water entering a boiler/water tank in order to reduce demand to heat water to a set temperature.
Recognised to be simple and effective ways to reduce energy demand heating, developers should consider both heat recovery options, especially in buildings predicted to have high heat demand.
How efficient is it?
Efficient MVHR units will operate at 88 – 90% heat recovery rates but are capable of recovering up to 95% of the heat in a building. MVHR is more efficient than natural ventilation and can be used for dwellings where noise and air pollution concerns exist, meaning windows cannot be opened. WWHR units are less efficient than MVHR units but are still approximately 55–60% efficient at recovering waste water heat.
Location and space requirements
It is essential that MVHR are accessible to carry out maintenance checks and to replace filters. The total height of the unit, sound-attenuating elements, manifolds and ducts will usually not exceed 2 metres. Throughout an average medium- sized house, ducts will not exceed 10cm and therefore do not require a significantly large space. Duct routing should however be carefully thought out to limit intrusion of ducts. Duct length should be as short as possible to mitigate heat loss risks.
WWHR units do not have any significant location or space requirements.
Things to be aware of (e.g. noise and visual impact)
MVHR can be noisy if not correctly installed, so appropriate acoustic treatment should be integrated. Systems should be designed into new buildings from the beginning, as the system should be present in every room in order to balance the ventilation requirements. Homes must be well insulated otherwise the efficiency losses could become costly.
Suitability/applicability across schemes
For both types of heat recovery, assuming the home is well insulated and has sufficient unit and duct space, suitability and applicability should be high. It will be increasingly difficult to integrate heat recovery systems the later they are considered in the design process.
Table 11:
Direct electric heating
What is it?
Direct electric heating uses panel heaters to heat internal spaces. It can also heat hot water being incorporated in the water fitting (e.g. instant hot water shower) and/or in an immersion hot water tank.
How efficient is it?
The technology is 100% efficient as each unit of electricity is directly converted into a unit of heat. <aqHowever, direct electric heating is approximately three times less efficient than any type of heat pump and therefore, relatively, is highly inefficient when compared to alternative technologies.
Location and space requirements
Direct electric heating panels will typically be located in the same way as a traditional radiator. Direct electric hot water can be incorporated in the water fitting (e.g. instant hot water shower) and/or in an immersion hot water tank. No major space requirements.
Things to be aware of (e.g. noise and visual impact)
Direct electric heating has minimal noise or visual negative impacts but these benefits are outweighed by high running costs and relatively low heating efficiency compared to heat pumps.
Direct electric heating is not generally desirable unless the building fabric thermal efficiency is exemplary and solar electricity generation is also provided to offset some of the running cost.
Suitability/applicability across schemes
Technically achievable for the majority of schemes but direct electric heating installations would make compliance with Policy NZC2(B) significantly more challenging due to the inferior efficiency compared to heat pumps. Due to the potentially high costs of operating direct electric heating, it should be avoided in developments where the occupants may be vulnerable to energy costs, such as social housing.
Table 12:
Energy storage
What is it?
An energy storage system allows heat or electricity to be captured when it is readily available, typically from a renewable energy system, storing it for use later. The most common energy storage systems include electric batteries, heat batteries and thermal stores.
Electricity can be stored in electrical batteries, or it can be converted into heat and stored in a heat battery. Heat batteries store spare electricity or heat by containing material which capture this energy by changing from liquid to solid. Heat can also be stored in a hot water cylinder as thermal storage. (See 'Domestic hot water storage'). Domestic-scale hot water storage is most common; however, larger communal hot water storage is also possible and can be effective in developments with large solar arrays or a shared heat network. When well-designed these can even deliver cross-seasonal heat storage, e.g. in an underground insulated tank.
Energy storage is useful for buildings that generate their own renewable energy, as it allows them to use more of their low carbon energy. Energy storage is seen as key to supporting the renewable transition, making best use of local energy resources, and supporting grid modernisation.
How efficient is it?
A typical residential solar system without a battery will cover about 30%-50% of household power consumption. With a battery system, this can be increased to 80%, 90% or potentially even 100% of household power consumption, by bridging the time gap between when solar energy is generated and when energy is used.
Location and space requirements
Battery storage units need to be in well-ventilated areas and away from sources of heat, including direct sunlight.
Units should be easily accessible for maintenance and should be enclosed and have suitable warning signs indicating that a large amount of energy is stored within.
In addition, batteries need to be able to be quickly isolated from the electricity network in the case of an emergency.
Batteries can be quite heavy so a structural engineer may be needed to review the storage location and recommend appropriate fittings to meet building regulations.
Things to be aware of (e.g. noise and visual impact)
Residential installations must be placed with consideration of proximity to sleeping and living areas and proximity to neighbouring buildings so it does not pose a risk to residents should the battery fail or catch fire.
Systems should be in an exterior location or in a garage or outbuilding. If placed on the exterior of the building they should be sensitively placed so as not to impact the visual amenity.
Suitability/applicability across schemes
Most suitable for homes that have installed Solar PV systems as they can help store excess generation and maximise the use of renewable energy.
Communal thermal storage is likely to be most effective in a development with a large solar PV array or shared networked heat.
Table 13:
Solar photovoltaic panels
How efficient is it?
Solar photovoltaic (PV) installations produce electricity from sunlight and can be mounted or integrated into the roofs or façades of buildings or may be installed on the ground. They are a common form of renewable energy that is considered mainstream within the building industry.
PV arrays now come in a variety of shapes and colours, ranging from grey ‘solar tiles’ that look like roof tiles to panels and transparent cells that can be used on conservatories. PVs can be used to provide extra power for buildings already connected to the national grid or can also provide the only source of electricity for a building. They can be combined with green roofs.
How efficient is it?
The majority of solar panels on domestic systems in the UK are around 10-20% efficient although some types of solar panels can reach an efficiency level of up to 25%.
However, note that this efficiency tells you how effective a solar panel is at converting sunlight into electricity. Whilst this might sound like a low figure, a solar panel system can generate enough electricity to dramatically reduce energy bills and carbon emissions
Location and space requirements
Space is a key consideration. The average system size is around 3.5kWp and this will typically take up around 20m2 roof area.
An unshaded, South facing roof is ideal for maximum electrical output. East or West facing roofs could still be considered, but North facing roofs are not recommended. A system facing East or West will yield around 15-20% less energy than one facing directly South.
However, a ‘concertina’ pattern (with panels alternating between east and west facing) enables a greater total area of PV panels to fit within the given area of roof, and therefore a greater concentration of total kW generation per square metre of roof space. This kind of configuration can help buildings with a small ratio of roof area to floor area (that is, taller buildings) to achieve the targeted renewable energy generation.
Any nearby buildings, trees or chimneys can shade roofs and have a negative impact on the performance.
Things to be aware of (e.g. noise and visual impact)
There are visual impacts to consider – rooftop solar PV installations may not be possible and heritage and conservation designations must be considered.
Generally, solar panels should not make noise, unless there is a structural defect or a problem with the installation.
Solar Panels can provide a space for birds, in particular pigeons to nest under. Consideration should be given to netting or mesh to avoid unwanted nuisance.
Suitability/applicability across schemes
Solar PV panels are considered ‘permitted developments’ and often don’t require planning permission. However, exceptions apply e.g. listed buildings and conservation areas.
Solar PV should be considered standard for new developments and the design of buildings should incorporate them. However, across the district care must be taken to ensure the visual impact is not adverse and there may be instances where they may not be appropriate.
Table 14:
Solar thermal
What is it?
Solar thermal uses the energy from solar radiation to heat a fluid within a collector (either a panel or tubes). The fluid can be water or a water-glycerol mix, which is then used in a hot water cylinder or thermal store to pre-heat domestic hot water. A boiler or immersion heater is often used to further heat the water or to top up the quantity of supply to meet demand.
How efficient is it?
Solar thermal system is considered to have efficiencies of between 70% and 80%19.
Variations in sunlight hours and solar radiation levels lead to seasonal variations in efficiency of these systems.
Often requires a top-up system as above and is required in addition to a space heating system.
The energy savings can lead to paying off the system within 12-20 years.
Location and space requirements
Most commonly installed on roofs, these can be installed anywhere (e.g.: at ground level, or on a garage roof) as the equipment is not heavy and not requiring additional structural/support requirements.
The main consideration is overshadowing, as the time the panels spend under direct solar radiation determines the efficiency of the system. Panels or tubes should be orientated between south east and south west to ensure that the most sunlight hits the panels/tubes.
Things to be aware of (e.g. noise and visual impact)
There are visual impacts to consider – rooftop solar thermal installations may not be possible and heritage and conservation designations must be considered.
Items for consideration also include ensuring systems are fully sealed and resistance to moisture and other weather, electrical safety, wind uplift and suitable fixings and structural loading (eg: snow collection).
You may require a larger hot water cylinder for the immersion heater top-up.
Suitability/applicability across schemes
In most cases, the system would be acceptable under permitted development, however restrictions may apply where a building is listed or in a conservation area.
It is most suitable for developments with high domestic hot water demand such as residential and hotel buildings.
Table 15:
Combined Heat & Power engines (CHP)
What is it?
An engine (gas, biomass or biogas powered) that creates electricity and also captures the heat energy created in the process. Therefore, simultaneously creates both heat and power.
When additionally providing cooling, the systems are known as CCHP (combined cooling heat and power) and would include absorption chillers.
It is thought that in future, hydrogen-fired CHP may also emerge.
How efficient is it?
Most efficient when there is a significant heat demand – the heat load typically requires a development with a minimum of 50 homes before CHP systems are considered to be efficient.
They must also be used constantly in order to be most efficient, so the CHP should be sized to meet the annual base heating demand. CHPs over-sized will be less efficient and produce higher emissions, so peaking plant to avoid these should also be considered.
The choice of prime mover that creates the outputs has an impact on the emissions created: commonly these are internal combustion engines and gas turbines. Gas turbines produce lower emissions and are more electrically efficient
Location and space requirements
Systems require a centralised energy centre with space for the engine, in addition to hot water tanks, distribution pipework also has to buried.
Things to be aware of (e.g. noise and visual impact)
Local air quality considerations – burning any fuel locally can impact the quality of local air, especially when the fuel is burned on site, for example in a centralised energy centre or boiler room.
CHP systems supplied with fossil gas should be avoided as these lock the development into fossil fuel use for longer.
Where CHP systems are proposed, these should be low emission versions by up to date standards and benchmarks.
Where CHP is proposed, the long term trajectory to reduce carbon emissions to zero must be considered, using SAP 10 carbon factors (or more recent versions).
Although hydrogen CHP may emerge in future, hydrogen is not yet a truly sustainable fuel, as its production currently either uses fossil fuel or takes multiple units of electricity to produce each unit of hydrogen, making it less efficient than simply using that electricity in its original state. However, technological innovation may solve this problem in future. Should hydrogen CHP proposals come forward, it will only be considered acceptable if it can be guaranteed that the hydrogen supply is not from fossil fuel (unless the resulting carbon is guaranteed to be captured and stored permanently) or produced more efficiently and sustainably than if the required electricity were instead used directly at the site.
Suitability/applicability across schemes
Can be installed in centralised energy centres as part of a district- wide strategy. Best suited to new build schemes with consideration of the technology from the outset.
Can use same heat distribution system as gas boilers – hot water >60 degrees with radiators. Can be considered complex systems because of the need to balance the generation of heat and electricity, and as such systems must be designed and installed in accordance with best practice guidance and by specialist engineers.
Could potentially lead to higher energy bills for residents, therefore not a viable option where fuel poverty is a risk.
Biomass
What is it?
Using plant-based fuels or waste organic matter as a fuel within a boiler. Considered a renewable energy technology where the fuel source can be rapidly replenished.
Biomass fuel sources can include logs, woodchips, agricultural waste, industrial biological residues, or specially created wood pellets. Typical household systems tend to use logs, woodchip or pellets. These may heat a single room via a stove, or can heat multiple spaces and appliances via a biomass boiler.
How efficient is it?
Efficiency is dependent on the fuel used for combustion and the moisture content of the fuel, which can range between 94% (pellets) and 80% (wet woodchips)20. Therefore, systems can offer a similar efficiency to gas boilers.
Although burning biomass releases CO2 to the atmosphere, this can be offset by the CO2 absorbed in the original growth of the biomass and/ or the growth of new biomass to replace it (e.g. replanting commercial woodland after logging). Where this sequestration is taken into account, biomass boilers sometimes claim emissions ~70% lower than those of conventional gas boilers. However, see caveats about responsible fuel sourcing.
Location and space requirements
Siting of the boiler flue has to be considered and therefore biomass is often not appropriate on small spaces.
Space is required for storage of the biomass feed fuel - the size of store required depends on both the size of the biomass boiler and the frequency of deliveries of the fuel/distance to the fuel source. For example, a garage-sized store may be required for a large house so the store required may not be insubstantial.
In addition, the store should be able to accommodate the required fuel for a worst-case-scenario winter, which would have higher demand.
Local transport networks must be considered, for their capacity to accommodate transport vehicles such as trucks/lorries for fuel deliveries.
In addition, the carbon emissions and particulate matter from deliveries (as well as the biomass combustion itself) should be part of the feasibility considerations for this technology.
For this reason, on-site solutions such as heat pumps are often more favourable, both in terms of local emissions affecting air quality, and also the associated carbon emissions.
Things to be aware of (e.g. noise and visual impact)
Local air quality considerations – burning any fuel locally can impact the quality of local air, especially when the fuel is burned on site in a centralised energy centre or boiler room.
Carbon emissions and particulate matter are released both in the fuel transport and operation of biomass boiler.
In the Warwick District Air Quality Management Areas (AQMAs) a limit has been set for annual and hourly nitrogen dioxide limits21. Biodiesel can also be used in some biomass systems but this is not recommended due to air quality implications as it composed of animal oils and fats.
The presence of and impact on local protected habitats and species (including birds and bats nesting/roosting in chimneys/ roofs/sheds) must be fully investigated prior to installation of any biomass plant.
Responsible sourcing of biomass woodchip/pellets must also be demonstrated in order to certify this will not be associated with deforestation or forestry systems that fail to replant logged areas. Preference should be given to fuels that are a by-product of other processes and are produced in the UK. If external flues are required, there may be additional considerations around visual impact on any surrounding areas designated for natural or manmade heritage protection.
Further information on open fires and stoves Defra have produced the following guide. You can also visit the HETAS website for help. (www.hetas.co.uk)
Suitability/applicability across schemes
Can be retrofitted into existing buildings which have fireplaces and/or gas boilers and use radiators as the heat distribution system.
Can be installed in centralised energy centres as part of a district- wide strategy, or can be designed to heat a single room or dwelling.
Pellets are easier to use and can run automatically with fuel feeders which refill the boiler when the fuel runs low. Log-burning boilers must be filled by hand and therefore require more maintenance and intervention.
Unlikely to be suitable for schemes in urban areas due to air quality impacts.
Table 17:
Wind
What is it?
Wind turbine blades are rotated in low to medium level wind, and this movement is used to generate electricity.
For electricity generation, small- scale wind generation is often considered a less viable solution than a PV array (so wind is more likely to be considered where large scale generation is possible).
<5kW micro turbines are most suitable at a building scale (with blade diameters up to 3m), whereas macro turbines can have a capacity up to 5MW.
How efficient is it?
In suburban areas for turbines at 10m in height and working at 10 metres/second, annual efficiency ranged between 20% and 40%.
Most useful in rural locations where electrical grid connection is less reliable or not available, where obstacles and availability of most suited wind conditions can improve the efficiency of standalone turbines.
Location and space requirements
Site context is crucial for deciding whether wind turbines are feasible, both for the siting of the turbines themselves and if the conditions are suitable.
Average wind speeds, required separation distances from neighbours, grid connection, site access and environmental and/or landscape designations all need to be considered.
Predominant winds and average wind speed to be analysed. The UK Wind Speed (NOABL) Database should be used as a baseline, but calculations should be modified to incorporate local geographical context (terrain and obstacles).
Separation distances from neighbours, and between turbines, where necessary, to be calculated. Best suited to rural locations due to the need to have undisturbed wind pathway to the turbines.
Can be operated in an off-grid manner or connected to local/ UK-wide electrical grids. Where operated off-grid will need to be installed in collaboration with electrical battery storage to store electricity when it is generated but not used, and also to stabilise supply and demand. Where connected to the grid, battery storage can also aid in the demand side response system.
Adequate and appropriate grid connection is required.
Site access for wind turbine construction and maintenance. Similarly, rooftop/facade access route and space is required where turbines are building-integrated.
Things to be aware of (e.g. noise and visual impact)
Considered to have potential noise impacts22, as turbine design develops, the noise concerns are becoming less of a barrier. Blade design has created low-noise options, in addition to vibration isolators, sound absorbing materials used for the gearbox and generator components can help reduce and minimise noise generation. Environmental Health may need to be consulted with regards to noise nuisance for neighbours.
Landscape and environmental designations will need to be fully considered and any impacts investigated. This includes local ecology and trees, local habitats (especially considering bird populations).
Historic environment and heritage views will also need to be considered.
Suitability/applicability across schemes
Building-integrated turbines can be used within new and existing developments.
Consideration must be given to wind speed and direction as these will impact the level of wind generation, and the number of hours the turbines can be operating at full capacity. For example, wind is strongest at night but local context will inform the number of hours the turbines can operate.
Planning permission may be required and will be subject to local and national policies relating to wind turbines.
Table 18:
Hydro
What is it?
Energy harnessed from falling or flowing water in rivers/dams, using kinetic energy to move turbines, create mechanical energy and drive a generator to create electrical energy.
Micro-hydro plant produce <50 kilowatts (kW), small scale plants between 50kW and 5 megawatts (MW) and large-scale plants >5MW23.
How efficient is it?
Efficiency of the hydro turbine is usually >80%, which is double that of a steam engine24.
The amount of hydroelectric power for a system is dependant on the vertical distance that the water falls (the 'head') and the level of water flow25 .
Location and space requirements
Local opportunities for hydropower may exist in areas with hills/waterfalls/weirs. Some local potential at weirs at Barford on the River Avon is identified in the 2021 Warwick Low Carbon Energy Feasibility report (although there may also be other local opportunities).
The availability of a suitable water source and adequate space to develop a hydropower plant mean the number of feasible sites are quite restricted. Many of the viable sites for hydro have been considered in the UK already.
Hydroelectric schemes in the UK typically are25:
- storage schemes (using a dam).
- pumped storage using electricity to pump water between two reservoirs. Water is pumped when the demand and electricity price is low (e.g.: at night) and water is released when demand and price are higher. May not be considered fully renewable as it requires electricity central to its operation.
- run-of-river hydro using a weir to enhance the natural river flow.
Things to be aware of (e.g. noise and visual impact)
Before a scheme can be built, the upfront and operational costs, environmental permits, planning consent and connection to local electrical grid must be considered. These are in addition to heritage and historic environment considerations.
An ecologist must be appointed, and local environmental/ conservation bodies must be consulted at early stages of development in order to fully consider potential environmental impacts on biodiversity, water wildlife and wider impacts from interrupting natural water flows; this includes consultation with the Environment Agency and Natural England.
Suitability/applicability across schemes
Please refer to the Warwick District Low Carbon Energy Feasibility Report (RINA Tech UK, June 2021).
The role of district heating and cooling networks
5.6 District heating (DH) and cooling networks supply thermal energy (heating and/or cooling) to multiple buildings, usually from a centralised heat source (energy centre), through a network of insulated pipes and heat exchangers. District heating (or heat networks) are 'energy source agnostic' meaning they can accommodate a range of heat pumps (both ground and air source) and waste heat from industry or commercial uses.
5.7 DH networks can be designed for new-build schemes as well as allowing the connection of existing buildings. They can supply heat at the same conditions as existing individual boilers, allowing internal heating systems to remain unchanged, however newly designed heat networks can use lower temperature sources that operate at lower temperatures and a wider delta T (the temperature difference between supply and return) compared to individual boilers. This translates to lower energy consumption, both in terms of heat losses and pumping energy, but connecting to these can be problematic for existing, older buildings as their existing internal heating systems may not be designed to emit heat at a lower temperature (and may therefore need to be upgraded to operate at this lower temperature if connecting to such a network).
5.8 Shared heating and cooling networks can also operate on a partly decentralised heat source, e.g. heat removed by active cooling from offices, refrigerated areas and ICT server rooms could be inserted into the network and transferred to where it is needed (e.g showers, laundries, etc). This is a key opportunity in mixed-use development, or residential development that takes place near existing large heat rejection, such as supermarkets, cold storage or ICT server farms.
5.9 As per Warwick Local Plan Policy CC2-Planning for Renewable Energy and Low Carbon Generation (point ‘e’) where possible, homes and buildings should maximise appropriate opportunities to address the energy needs of neighbouring uses and should link to existing or planned local carbon district heat networks.
5.10 DH networks are often most beneficial for new development sites and in areas where there is a high energy demand density. Therefore, town centres or larger new-build masterplans are ideal locations due to the range of use classes and densities. However, heat networks can also be beneficial in rural, off gas areas where homes are currently reliant on more volatile energy source such as oil and LPG. Examples include the Swaffham Prior heat network in Cambridge that could connect up to 300 homes. The Swaffham Prior network will be fed by heat pumps (a combination of air-source and ground-source). In this instance the switch to low carbon energy sources and network can have wider benefits such as reducing instances of fuel poverty.
5.11 Where DH networks are specified, it is critical that all heat networks provide affordable, reliable year- round, hot water and heating and ideally also cooling where there is a cooling demand. Heat networks must demonstrate compliance with appropriate technical standards (currently CIBSE's Heat Networks CodeofPractice for the UK) and be registered with the Heat Trust.
5.12 Consideration must also be given to the installation of the pipe networks and other wider development plans in the area and the potential impact on local landscape and biodiversity due to the scale of works required.
5.13 As per NZC2(B) of the Net Zero Carbon DPD, where DH networks are proposed, applications should be accompanied by an energy statement that includes an assessment of the advantages of a network system vs individual systems, an accurate assessment of distribution heat losses, a long term strategy for the sustainable supply of low carbon fuel and that the network has a credible route towards achieving zero carbon status.